

Moreover, 239Pu and 240Pu cannot be chemically distinguished, so expensive and difficult isotope separation would be necessary to separate them. (However, in modern nuclear weapons using neutron generators for initiation and fusion boosting to supply extra neutrons, fizzling is not an issue.) It is because of this limitation that plutonium-based weapons must be implosion-type, rather than gun-type. As a result, plutonium containing a significant fraction of 240Pu is not well-suited to use in nuclear weapons it emits neutron radiation, making handling more difficult, and its presence can lead to a " fizzle" in which a small explosion occurs, destroying the weapon but not causing fission of a significant fraction of the fuel.
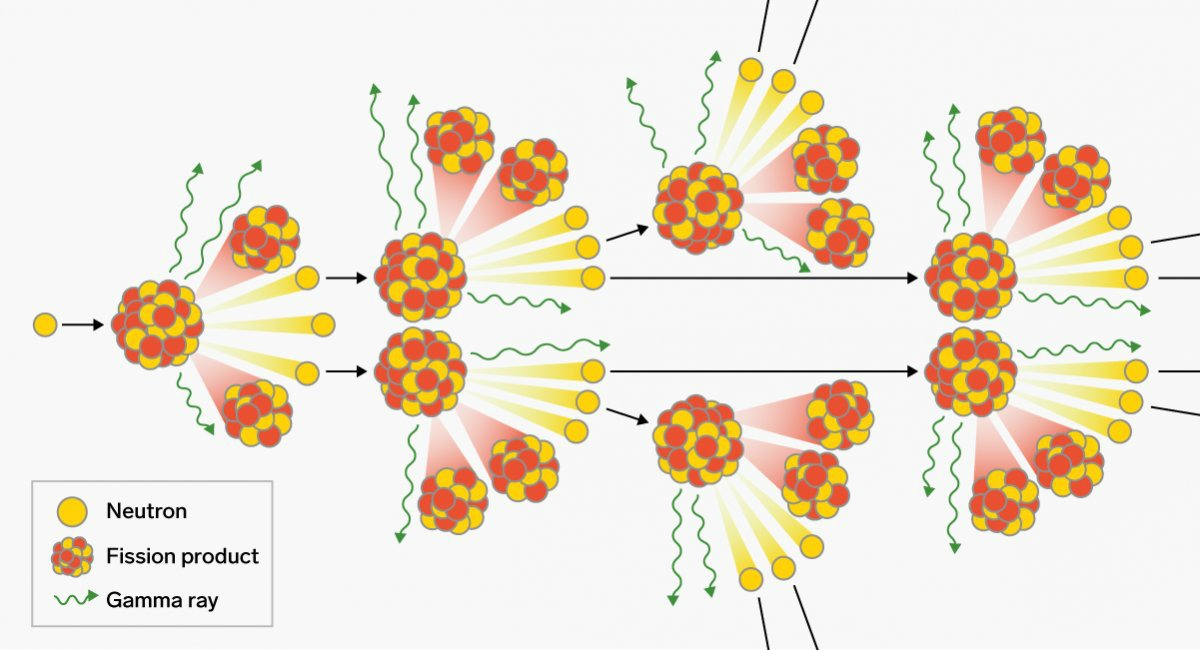
240Pu has a high rate of spontaneous fission events (415,000 fission/s-kg), making it an undesirable contaminant.

In practice, however, reactor-bred plutonium will invariably contain a certain amount of 240Pu due to the tendency of 239Pu to absorb an additional neutron during production. kg), making it feasible to assemble a mass that is highly supercritical before a detonation chain reaction begins.Pure 239Pu also has a reasonably low rate of neutron emission due to spontaneous fission (10 fission/s Only if the fuel has been exposed for a few days in the reactor, can the 239Pu be chemically separated from the rest of the material to yield high-purity 239Pu metal.Ģ39Pu has a higher probability for fission than 235U and a larger number of neutrons produced per fission event, so it has a smaller critical mass. The 239U then rapidly undergoes two β − decays - an emission of an electron and an anti-neutrino ( ν ¯ e įission activity is relatively rare, so even after significant exposure, the 239Pu is still mixed with a great deal of 238U (and possibly other isotopes of uranium), oxygen, other components of the original material, and fission products. This happens more easily with lower kinetic energy (as 238U fission activation is 6.6MeV). Occasionally, when an atom of 238U is exposed to neutron radiation, its nucleus will capture a neutron, changing it to 239U. 239Pu is normally created in nuclear reactors by transmutation of individual atoms of one of the isotopes of uranium present in the fuel rods. Total heat released in a thermal-spectrum reactor (anti-neutrinos do not contribute) Radiation source (thermal fission of 239Pu)Įnergy released by radiative capture of prompt neutrons 19.98 TJ/ mol = 83.61 TJ/kg, or about 23 gigawatt hours/kg. The fission of one atom of 239Pu generates 207.1 MeV = 3.318 × 10 −11 J, i.e. Using appropriate triggers, neutron reflectors, implosion geometry and tampers, the critical mass can be less than half of that. A spherical untamped critical mass is about 11 kg (24.2 lbs), 10.2 cm (4") in diameter. Of all the common nuclear fuels, 239Pu has the smallest critical mass. Plutonium-239 can also absorb neutrons and fission along with the uranium-235 in a reactor. The fissioning of an atom of uranium-235 in the reactor of a nuclear power plant produces two to three neutrons, and these neutrons can be absorbed by uranium-238 to produce plutonium-239 and other isotopes. The nuclear properties of plutonium-239, as well as the ability to produce large amounts of nearly pure 239Pu more cheaply than highly enriched weapons-grade uranium-235, led to its use in nuclear weapons and nuclear power plants. Plutonium-239 has a half-life of 24,110 years. Plutonium-239 is also one of the three main isotopes demonstrated usable as fuel in thermal spectrum nuclear reactors, along with uranium-235 and uranium-233. Plutonium-239 is the primary fissile isotope used for the production of nuclear weapons, although uranium-235 is also used for that purpose. Plutonium-239 ( 239Pu or Pu-239) is an isotope of plutonium.
